Mineral texture and composition of magnetite in the Sahavaara IOCG deposit, Pajala area: a mineral processing approach
Full text
(2) Mineral texture and composition of magnetite in the Sahavaara IOCG deposit, Pajala area - A mineral processing approach.
(3) Abstract Stora Sahavaara is one of several iron (Cu-Au) deposits of economic potential in the PajalaKolari area and it is currently developed for mining by Northland Resources Inc. These deposits are hosted by 2.3-2.0 Ga Karelian greenstones and occur as magnetite-rich lenses at certain stratigraphical positions and often in association with carbonate rocks. They contain various amounts of skarn minerals with serpentine, pyroxene, and amphibole as the most important gangue minerals. The Stora Sahavaara iron occurrence was previously considered as belonging to a broad group of magnetite-dominated, Ca-Mg- and Mg-silicate skarn-hosted deposits, which occur throughout northern Sweden, within a Karelian volcano-sedimentary domain. Several deposits considered to be of the same type also occur and have been well studied in the Finnish part of the Lappland, in the Kolari district. These deposits, which are interpreted to be epigenetic Fe-Cu + Au deposits, occur within the same structures as the Stora Sahavaara deposit. They are suggested to belong to the increasingly important group of iron-oxide-copper-gold (IOCG) deposits. A characteristic feature of the Stora Sahavaara magnetite is the high Mg content of its concentrate which is suggested to be lattice bound. In some cases the recovery (magnetite) is poor, or the concentrates are not clean, which is clearly indicated by a high SiO2 content in the concentrate. Also, significant inclusions/intergrowths of sulphides in magnetite grains could cause the final iron ore concentrate to have high S content, for example. Alternatively, the grade may be lower than predicted due to silicate or other gangue carrying finely disseminated magnetite in some textural ore types. The general purpose of this work is to apply the mineralogy and geology of the Stora Sahavaara deposit in order to better understand the metallurgical processing characteristics of the ore. For the mineral processing of the ore and the properties and quality of the final products, the texture of magnetite, its impurities and its chemistry is of great importance. According to the data presented, the principal ore minerals of the Stora Sahavaara magnetite deposit are Mgt-Po-Py-Ccp. The main ore mineral magnetite contains high amounts of Mg, bound to the crystal lattice only in cases where coarse-grained textures of the first generation occur and where serpentine skarn dominates. The dominant sulphide is pyrrhotite which exists in both monoclinic and hexagonal crystal forms. The monoclinic crystal form of pyrrhotite is the principal factor behind some S-bearing concentrates as it turns to report with magnetite. All other metal oxides such as SiO2+Al2O3, TiO2 and vanadium, which have upper acceptable limits in the Direct Reduction Ironmakers (DRI) pellets, which is the pellet towards which Northland’s efforts are primarily directed, are within acceptable ranges. This study shows that the Stora Sahavaara magnetite composition is suitable for the DRI type of pellets most especially considering blending. It gives a detailed characterization of the magnetite, and will hopefully serve as a useful manual during the mining process.. ii.
(4) Acknowledgements My heartfelt thanks goes to the ―market and man all master‖ (God) for not only giving me the opportunity to be hear and pursue studies, but also for his protection and guidance throughout my studies and the favour from all with whom I came in contact. I am very grateful to Northland Resourses Inc for funding this project. Special thanks to Gustav Hallabro and the entire Pajala staffs for the warm reception they gave me. I want to thank my supervisors; Dr. Olof Martinsson (LTU), Johan Högnas and Petri Peltonen (Northland Resources) for sharing their experience with me and putting in place all the materials used to accomplish this project. I say thank you to Lawrence for initiating this project. My profound gratitude goes to Bo Arvidson and Dr. Christina Wanhainen for their constructive suggestions on the manuscript. I want to single out Prof Lennart WILDENFALK and Dr Anders WILDERLUND for without them being devoted this program should have been a mere dream; you guys are role models of this generation. I am also thankful to all the teachers of the Department for their instructions and knowledge in the course of this program. Special thanks to my perents Mr Tanteh ABONGWA John and Mme Mary Etukene, I am short of words for without your love and proper upbringing right from my childhood days I possibly won`t have been such an resourceful mine today. To my brothers and sisters, you guys have been really wonderful, for without your encouragements and understanding things will not have been this positive. Exceptional thanks to my dearest Pascaline MANKA for always standing by me all through this rigorous period of my life. Elvis Tangwa I say thank you for all this years we lived together like brothers. Let me use this opportunity to wish you success in all your life endeavours. To Mr Gemain MUKURI and family I am very grateful for the big brotherly and fatherly love you shown to me all this years. Life will have been a lot more different without you always being there for me. I want to thank Carine and Honorine Elambi for being such a great comforter when things seem to be nonexisting. To all of my friends both home and abroad I say thank you and God bless you all. Every seconds spent on this project has been challenging and wonderful. This project took me to places I never thought of and my skills into a new direction and level. I am very delighted I stood the challenges of time and realise this project thanks to the support I received from Northland Resources Inc. Luleå, October, 14th 2009. Paul .W. ABONGWA.. iii.
(5) Table of Contents Abstract ..................................................................................................................................... ii Acknowledgements .................................................................................................................. iii General Introduction ............................................................................................................... 1 CHAPTER I: GENERALITIES ............................................................................................ 2 I.1Thesis Objectives ............................................................................................................... 2 Main Objectives .................................................................................................................. 2 Metallurgical Objectives ..................................................................................................... 2 Specific Objectives ............................................................................................................. 2 I.2 Methodology ..................................................................................................................... 3 I.3 Review of Research ........................................................................................................... 4 3-a) IOCG Deposits (synthesis) .......................................................................................... 4 Definition ........................................................................................................................ 4 3-b) Characteristics ............................................................................................................. 7 Hydrothermal features ..................................................................................................... 7 Mineralization and time-space patterns ........................................................................... 7 Morphology and Tectonic settings .................................................................................. 8 Economic Characteristics ................................................................................................ 8 Geophysical features ....................................................................................................... 9 3-c) Ore genesis .................................................................................................................. 9 3-d) Exploration Implications ........................................................................................... 12 I.4 Area of study and Exploration history ............................................................................ 12 4-a) Location of study area ............................................................................................... 12 4-b) Exploration history and Review of previous work in the area .................................. 14 Exploration history ........................................................................................................ 14 Review of previous work .............................................................................................. 15 I.5 Regional Geology ............................................................................................................ 16 I.6 Deposit (district) Geology ............................................................................................... 18 CHAPTER II: PETROGRAPHIC AND MINERALOGICAL DESCRIPTIONS .......... 22 II.1 Macroscopic observations .............................................................................................. 22 II.2 Microscopic observations .............................................................................................. 24 2-a) Foot and Hanging wall Rocks: .................................................................................. 24 SAH63008 ..................................................................................................................... 24 iv.
(6) SAH65005 ..................................................................................................................... 25 SAH63021 ..................................................................................................................... 27 II.3 Microscopy of Ding Davies Tube (DDT) Test Buttons................................................. 28 II.4- The Ore (Combined microscopic and DDT Observation) ............................................ 30 4-a) Northern Terminal ..................................................................................................... 32 4-b) Central Zone .............................................................................................................. 34 4. c) Södra Sahavaara ........................................................................................................ 37 CHAPTER III: CHEMICAL ANALYSES, METALLURGICAL AND PROCESSING IMPLICATIONS. ................................................................................................................... 39 III.1 CHEMICAL ANALYSIS OF ORE AND WALL ROCKS.................................... 39 - Relationship of ore, wall rocks and the different skarn types. .................................... 49 III.2 METALLURGICAL AND PROCESSING IMPLICATIONS ............................. 51 2-a) Analyses of DDT tested concentrate ......................................................................... 51 A-i) Northern Terminal ..................................................................................................... 51 SAH05004: .................................................................................................................... 51 SAH07005: .................................................................................................................... 54 SAH07006: .................................................................................................................... 57 SAH05011: .................................................................................................................... 60 SAH07021: .................................................................................................................... 63 SAH07021B: ................................................................................................................. 66 A-ii) Central zone .......................................................................................................... 69 SAH05012: .................................................................................................................... 69 SAH05008: .................................................................................................................... 72 SAH07022: .................................................................................................................... 75 SAH05013: .................................................................................................................... 78 SAH05014: .................................................................................................................... 85 SAH06002: .................................................................................................................... 88 A-iii) Southern Terminal ............................................................................................... 91 SAH07014: .................................................................................................................... 91 SAH07015: .................................................................................................................... 94 SAH07011: .................................................................................................................... 96 A-iv) Södra Sahavaara .................................................................................................. 99 SAHO7019: ................................................................................................................... 99 SAH07020: .................................................................................................................. 102 v.
(7) 2-b) Microprobe Analysis of Magnetite ......................................................................... 112 B-i) Northern Terminal: .............................................................................................. 112 B-ii) Central Zone ....................................................................................................... 116 CHAPTER IV: GENERAL DICUSSION AND INTERPRETATION .......................... 121 CHAPTER V: CONCLUSION AND RECOMMENDATIONS ..................................... 125 V-1 CONCLUSION ........................................................................................................... 125 V-2 RECOMMENDATIONS ............................................................................................ 126 References Appendices. vi.
(8) General Introduction Northern Sweden has been known for centuries for its enormous endowment of mineral resources. Since the discovery of the Iron-oxide copper gold (IOCG) type of deposits by Hitzman et al. (1992), northern Sweden has been revisited in several aspects such as geology, mineral deposit model, metallogeny, as well as the geophysical aspects. The present study goes a step further to the metallurgical and processing aspects. Several of the formerly discovered deposits have been re-studied and some have been reclassified to belong to the IOCG class of deposits. Other types of iron deposits in this region, most especially the Kiirunavaara apatite iron ore deposit (AIO) are still pending consideration to be defining end members of the IOCG style of mineralization. After the classification by Hitzman et al. (1992), iron-oxide deposits have gain exploration and research interest worldwide. These types of deposits exhibit large diversity in terms of hydrothermal features, mineralisation and time-space patterns, as well as in their geophysical features, and they are important sources for copper and gold. However, contradiction in possible genetic links between IOCG and Cu deposits exists. Sweden is the largest exporter of iron ore in Europe (fig 1). The northern Norrbotten region has for long been known for hosting some of the world’s largest apatite-iron ores (Kiirunavaara and Malmberget). Recent consideration of IOCG deposits in northern Sweden is the Kaunisvaara field in the Pajala region consisting of eight separate ore bodies, from N→S: Palotieva, Norra Tapuli, Stora Tapuli, Stora Sahavaara, Södra Sahavaara, Suksivuoma, and Karhujärvi. Five of these deposits, Palotieva, Norra Tapuli, Stora Tapuli, Stora Sahavaara, and Södra Sahavaara, were discovered by V Tanner in 1918. The Ruutijärvi deposit was discovered 31 years later (1949) by the Boliden Mining Co., and the Suksivuoma and Karhujärvi deposits were discovered by Johnson and Co. The Sahavaara deposit is the largest and economically most important of the ores found till date in this part of northern Scandinavia (Lundberg 1967). A majority of these deposits are 100% owned by Northland Resources Inc (formerly North American Gold Inc). The present study is concerned with the Stora Sahavaara deposit, one of Northland`s principal assets. The economic aspect (size and mineral content) of this deposit makes it one of few such projects in Europe. The final resource estimation considering a 25 % Fe cut-off grade is 77.036 x 106 tonnes at 43.3 % Fe and 0.08 % Cu; measured, 44.635 x 106 tonnes at 43.3 % Fe and 0.08 % Cu; indicated, and an inferred resource of some 23 million tonnes at 42 % Fe and 0.05 % Cu. (Northland Technical report, 2006). The total resource estimate thus stands at 1.45 x 108 million tonnes and a total reserve of 1.22 x 108 million tonnes. One problem with the Stora Sahavaara ore is the nature of its concentrate as revealed by metallurgical tests carried out in 2007. This is clearly indicated by a significant MgO and SiO2 content in the concentrate. In some cases also the recovery is poor. The grade may be low due to silicate or other gangue carried within magnetite in some textural ore types. As this mining project is already in an advance stage, this piece of work will hopefully be a useful guide-line in. 1 Mineral texture and composition of magnetite in the Sahavaara IOCG deposit, Pajala area -. A mineral processing approach.
(9) applying mineralogy and geology for predicting or and better understand metallurgical processing characteristics of the ore.. CHAPTER I: GENERALITIES I.1Thesis Objectives Main Objectives The general objective of this study is to investigate the possibility to apply mineralogy and geology for prediction, and/or better understanding of metallurgical processing characteristics of the Stora Sahavaara ore. With systematic drillcore logging and sampling of textural and paragenetic variations of the ore, and a good spatial distribution, it is possible to identify zoning patterns within the deposit. Chemical and textural-paragenetic variation of magnetite is of high importance for mineral processing and metallurgical purposes. (The basis of this thesis is not to give a complete spatial model; nevertheless, it should target the basis and ideas which can be use in achieving such a model.) Metallurgical Objectives The only significant metallurgical data available are magnetic separation tests (Dings Davis Tube tests) of ca. 200 drill core samples from Stora Sahavaara and reports by SGS covering the metallurgical implications. High amounts of inclusions and intergrowths of sulphides in magnetite grains could also be a problem as this will result in high S content in the final iron ore concentrate. Silicates or other gangue carried within magnetite may lower the grade of the ore. Another concern is the high MgO concentration in magnetite, which appears to be mostly in the magnesioferrite-magnetite solid solution. This MgO will appear in the final magnetite concentrate. Therefore, in order to improve grade control, some basis for predicting and modelling the MgO distribution in the ore should be developed. Specific Objectives Optical reflective light microscopy of magnetic and nonmagnetic fractions for paragenetic and textural interpretations. Characterisation of sulphides in the magnetic and nonmagnetic fractions, proportions in different fractions? Characterisation of the pyrrhotite; monoclinic or hexagonal? Characterisation of magnetite in the nonmagnetic fraction; grain size? Intergrowth with silicates? Characterisation of magnetic fractions with high SiO2 content; which silicates are present? Why did they enter into magnetic fraction? How does MgO content of magnetite correlate with assay and logging data; can we predict the MgO content in the concentrate from either of these?. 2 Mineral texture and composition of magnetite in the Sahavaara IOCG deposit, Pajala area -. A mineral processing approach.
(10) I.2 Methodology The first part of this work involved optical light microscopic study of thirty nine (39) DDT buttons at the Microscope laboratory of Luleå University of Technology (LTU). Both the magnetic and nonmagnetic fractions were considered. The samples were selected to be representative of the Stora Sahavaara ore body (appendix 1). A total of 233 DDT tests have been performed so far on the Stora Sahavaara and Södra Sahavaara deposits. The DDT sample particle size is ca. 80 % passing 75 µm. The aim of the light microscopic study on the DDT buttons was to evaluate the DDT test results and find possible reasons for the poor quality of some of the DDT concentrates in previous studies. Petrographic studies consisting of drillcore logging (21 drill core sections) and thin section work was followed by a brief field visit. During the logging process suitable samples were systematically selected for thin section. These samples were selected based on the general geology, the change in ore and silicate mineralogy, ore mineral paragenesis, textural variations, and on earlier achieved Dings Davis tube test results on this deposit. These samples were chosen to best represent different ore types. After macroscopic study of hand specimens, samples were sent to Canada where 70 thin sections were produce by VANCOUVER PETROGRAPHICS LTD. These thin sections were studied in detail at the LTU microscope laboratory using a standard petrographic microscope (Nikon ECLIPSE E600 POL). After close examination of the thin sections microscopically, suitable magnetite grains for microprobe analysis were then selected based on the following criteria; -. Change in silicate mineral composition, on the basis of: + How mixed they occur + How uniform they occur, i.e. solely serpentine, amphibole, pyroxenes and /or others + State of alteration of these silicates + Paragenesis of these silicates + Their relation with ore minerals and accessory minerals. - Ore mineral paragenesis and relationship with other minerals - Textural variation, and - Mineralisation style. The microprobe analysis were performed at the Geological Survey of Finland Research Laboratory at Espoo (Southern Finland Unit) using the CAMECA SX100 electron microprobe instrument. Analysing conditions were 20kV, 60nA, matrix correction by PAP method, and wave length dispersive spectrometers (TAP, LIF, PET diffracting crystals used). Before analysing, thin sections were coated with graphite. The graphite coating was done using a Japanese Electron Optical Laboratory (JEOL) JEE-4X Vacuum Evaporator. Bulk chemical analyses of rocks were performed by Dr Olof Martinsson (LTU). Analytical methods used are inductively coupled plasma mass spectroscopy (ICP-MS) and INA.. 3 Mineral texture and composition of magnetite in the Sahavaara IOCG deposit, Pajala area -. A mineral processing approach.
(11) I.3 Review of Research 3-a) IOCG Deposits (synthesis) Definition Iron oxide copper-gold (IOCG) deposits encompass a wide spectrum of sulphide deficient low-Ti magnetite and/or hematite ore bodies of hydrothermal origin where breccias, veins, disseminations and massive lenses with polymetallic enrichments (Cu, Au, Ag, U, REE, Bi, Co, Nb, P) are genetically associated with, but either proximal or distal to large scale continental, A- to I-type magmatism, and crustal-scale fault zones and splays. The deposits are characterized by more than 20% iron oxides. Their lithological hosts and ages are nondiagnostic but their alteration zones are, with calcic-sodic regional alteration superimposed by focused potassic and iron oxide alterations. The deposits have formed at shallow to mid crustal levels in extensional, anorogenic or orogenic, continental settings such as intracratonic and intra-arc rifts, continental magmatic arcs and back-arc basins. Margins of Archaean craton where arcs were developed appear to be particularly fertile. (Louise Corriveau, 2006) Currently known metallogenic IOCG districts (Fig. 1) occur in Precambrian shields worldwide and in Circum-Pacific regions (e.g. Carlon, 2000; Fourie, 2000; Porter, 2000, 2002a; Strickland and Martyn, 2002; Gandhi, 2004c; Goff et al., 2004). IOCG deposits recognition came into limelight by the discovery of the Olympic Dam deposit in 1975 (Roberts and Hudson, 1983) and was enhanced by the discovery of Starra (1980), La Candelaria (1987), Osborne (1988), Ernest-Henry (1991), and Alemao (1996). Further research by Hitzman et al., 1992 on early discoveries such as the Olympic Dam, defines these groups of deposits as the IOCG deposit type. This triggered re-classification of existing deposits, and discovery of new deposits. However, the diverse character of these deposits led to growing debate whether they form a single deposit type or are iron oxide-rich variants of other deposit types. There is also diverging opinions on their genesis and classification schemes (Table 1).. 4 Mineral texture and composition of magnetite in the Sahavaara IOCG deposit, Pajala area -. A mineral processing approach.
(12) Fig 1: Global distribution of Major IOCG and related type of deposits (Åsa Edfelt, 2007 PhD Thesis).. 5 Mineral texture and composition of magnetite in the Sahavaara IOCG deposit, Pajala area -. A mineral processing approach.
(13) Table1: Classification of magmatic-hydrothermal iron oxide deposits and related Cu Au deposits (after Gandhi, 2003, 2004a). →. Proximal → Distal Calc-alkaline magma Iron Skarn -Type Kiruna -type Olympic Dam -Type Cloncurry-type - Massive magnetite- Massive - Breccia(one or - Hydrothermal garnet-pyroxene magnetite-apatitemore stages), veins and actinolite magnetitedisseminations hematite matrix in older - Stratabound lensoid - Tabular, pipe-like `ironstones´ and irregular bodies and irregular - Pipe-like and and FeOx at intrusive contacts bodies, dykes and irregular bodies, mineralisation veins vent or fault controlled - Stratabound, - Monometallic Fe - Monometallic Fe breccias or and related FeOxand related Cu- Polymetallic: fault controlled Cu-Au deposits FeOx porphyry Fe, Cu, Au, Ag, deposits REE - Polymetallic: - Alteration: Sodic Cu, Au, Ag, - Alteration: Sodic - Alteration: - Magnitogorsk Bi, Co, W Potassic deposit, Russia - Alteration: - Kiirunavaara - OLYMPIC Dam Potassic deposit, Sweden deposit, Australia - Osborn and Starra deposits, Australia Source → Proximal → Distal Alkaline-carbonatite magma Phalaborwa-type Bayan Obo-type Source. -. -. Within or marginal to intrusion Veins, layers, disseminations and aggregates; late intrusive phase Low Ti magnetite, apatite, olivine, phlogopite, carbonate, fluorite, Cu sulphides, pyrites, PGE, Au, Ag, uranothorianite, baddeleyite Zoning in Ore; Sodic and potassic alterations Phalaborwa deposit, RSA. -. -. -. Hosted by country rock Veins, layers, disseminations and aggregates, startabound lenses Magnetite (replacive and /or pre-existing), hematite, bastnaesite, phlogopite, Fe-Ti-Cr-Nb oxides, fluorite, monazite, carbonate Zoning in ore; Sodic and potassic alterations Bayan Obo deposit, China. 6 Mineral texture and composition of magnetite in the Sahavaara IOCG deposit, Pajala area -. A mineral processing approach.
(14) 3-b) Characteristics Studies on all shortlisted IOCG deposits have shown that no set of geological features apart from the abundance of Cu and Au themselves readily distinguished economic Cu (-Au) systems (Olympic Dam or Candelaria types) from large accumulations of magnetite or hematite containing only anomalous amounts of these metals (Kiruna type or barren ironstones). Indeed, all regions containing significant Cu (-Au) deposits also contain numerous "barren" Fe-oxide-rich occurrences, thus regardless of its meaning, this empirical association provide the broadest signature for prospective regions (Barton & Johnson 2004). Hydrothermal features It was noted that although the exact alteration mineralogy within individual deposits depends on host lithology and depth of formation, there is a general trend from sodic alteration at deep levels, to potassic alterations at intermediate to shallow levels, to sericitic (hydrolytic) alteration and silisification at very shallow levels. The later are very restricted in space, thus commonly very close to mineralisation. Host rocks are intensely altered. Alteration zones particularly the early sodic and sodic-calsic style of alteration are extremely large (10`s to hundreds of sq. km) (Hitzman et al. 1992; Barton and Johnson 1996). It is readily recognised even in very high-grade metamorphic terrains were other evidences are lost. The mineralogy, relative development, and geochemistry of alteration types correlate with igneous rock compositions (Barton and Johnson, 1996). Skarn assemblages form in some deposits, with Mg-skarn forming within and/or close to the ore, while Ca-Mg skarn usually occurring further outside the ore. If Ca-rich host-rocks are present, Fe-rich garnet-clinopyroxene ± scapolite skarn assemblages may form (e.g. Heff prospect, British Columbia; Candelaria, Chile; Kiruna, Sweden; Shimyoka and Kantonga prospects, Zambia; Ray and Lefebure, 2000; Ray and Webster, 2000). Na (+/-Ca) alteration mobilize and typically remove base metals and ferrous metals from the rocks (Dilles and Einaudi 1992, Williams 1994, Johnson 2000). Barton and Johnson (2000) distinguish two broad types of K-metasomatism; a biotite or K-feldspar metasomatism common with calcic phases such as amphibole or clinopyroxene which is typically associated with introduction of magnetite/hematite ± Cu/Au, REE and other elements, and a second type of K-silicate alteration that is recognize in some young systems is oxidized (Hm stable), Kfeldspar-dominated (typically >8% K2O), and have a lateral extension of 10s of km. This second type is considered to depict recharge zones in certain continental and transitional marine settings. Mineralization and time-space patterns This class of deposit is characterized by abundance of iron oxide minerals and a relative lack of iron sulphides. Both the magnetite-apatite deposits and the iron oxide –Cu-Au deposits may contain significant carbonates, Ba, P, and F. The ore fluids responsible for the magnetiteapatite deposits appear to have been relatively saline, oxidised, sulphide-poor, aqueous fluids with temperatures above 250 0C. Formation of the Iron oxide-Cu-Au deposits appears to had involved either retrograde reactions of the fluids responsible for the magnetite deposits or fluid mixing with a separate, saline, oxidised, sulphate-bearing, lower temperature fluid, common with significant CO2 (Hitzman et al., 1992).. 7 Mineral texture and composition of magnetite in the Sahavaara IOCG deposit, Pajala area -. A mineral processing approach.
(15) The IOCG deposits may contain a suite of minor metals including U, Ag, Mo, Co, As and Zn; trace metal content is probably controlled by the surrounding host rocks. Almost all of the deposits of this class contain anomalous concentrations of REEs. Economic mineralization is dominated by paragenetically late chalcopyrite ± bornite and occurs within or near Fe-oxide accumulations (Barton & Johnson, 2004). Magnetite forms earlier at greater depth and/or at higher temperatures whereas hematite forms more distally and at shallow depth. The main ore stage is associated with potassium-iron alteration zones in which either sericite or K-feldspar prevail as the potassic phase (Skirrow et al., 2002; Belperio and Freeman, 2004). The ore mineral phases vary considerably among the deposits (Ray and Lefebure, 2000). Hitzman 2000 noted that very few deposits have economic Cu-Au mineralization and are typically interpreted to be late in the regional development. The lack of significant concentrations of Cu and Au mineralisation in some deposits may be due to the absence of suitable traps. Both local and regional mineralized zones correlate with major regional structural features and/or with volcano-plutonic structures (Barton & Johnson, 2004). Deposits are found in postArchaean rocks and are known from early Proterozoic to Pliocene. There is no specific time that appears more favourable for the iron oxide-Cu-Au deposits rather than the magnetiteapatite deposits. Morphology and Tectonic settings Deposits display a variety of morphologies from strata bound sheets to irregular stockwork breccia zones, veins, stratiform or discordant deposits and disseminated to massive lenses. Virtually all of the deposits form by replacement of host rocks. Rarely ore fluids appear to have reached the surface or form iron oxide sinters (Hitzman; 2000).There appears to be three ―end member‖ tectonic environments that account for the vast majority of these deposits: i. Intra-continental orogenic collapse; ii. Intra-continental anorogenic magmatism; and iii. Extension along a subduction-related continental margin All of these environments have significant igneous activity probably related to mantle underplating, high heat flow, and source rocks (sub-aerial basalts, sediments, and/or magmas) that are relatively oxidized; many districts contain evaporates (Hitzman; 1992, Adshead-Bell, 1998; Partington and Williams, 2000; Williams and Skirrow, 2000; Sillitoe, 2003). IOCG deposits are structurally control. The intersection of highly permeable units with fault zones in some deposits exerted a favourable control on ore deposition, while in others, dilational jogs, duplexes, splays on faults and shears, folding, or complex intercalation of high and low permeability, competent and incompetent units influenced fluid flow regime and position of alteration zones, breccias and/or ore deposition (Cross et al., 1993; Marschik et al., 2000; Partington and Williams, 2000; Skirrow, 2000; Davidson et al., 2002; Grainger et al., 2002; Sillitoe, 2003; Sleigh, 2002; Marshall, 2003).. Economic Characteristics Resource estimation from several IOCG deposits across the globe shows enormous geological resources with significant reserves of base, precious and other metals. They are major sources of Fe, Cu, Au, U, REE (LREE), F, vermiculite, significant sources of Ag, Nb, P, Bi, Co; and 8 Mineral texture and composition of magnetite in the Sahavaara IOCG deposit, Pajala area -. A mineral processing approach.
(16) sources of various by-products including PGE, Ni, Se, Te, Zr. They also contain several associated elements, notably As, B, Ba, Cl, Co, F, Mo, Mn, W, (Pb, and Zn). Resources for individual commodities are as high as some of the best examples of VHMS Au deposits in Canada and porphyry Cu worldwide (Louise Corriveau, 2006). The low gold values are not to be underestimated as the large tonnage of IOCG deposits compensates for the very low grades of all the metals present (Hitzman, 2000). Geophysical features The most readily applied geophysical techniques are magnetic, gravimetric, electrical, and radiometric. The abundance of iron-oxides, the irregular presence of sulphides, and the widely developed hydrothermal alteration rendered these methods suitable enough for this deposit type (Smith 2002). When interpreted in combination with geologic constraints, gravity and magnetic signatures reflect the distribution of Fe-oxide-rich bodies, as well as imaging district to regional-scale structural and igneous features (e.g., Gow et al. 1993, Haynes 2000). However, these geophysical techniques can produce quite complicated results in very complex settings making interpretation not quite ―text book‖. 3-c) Ore genesis A couple of genetic models for IOCG systems were suggested and after serious research and deliberations, two large families were considered based on fluid sources; magmatic and nonmagmatic fluid sources (Table 2). Saline, sulphide-poor, and relatively oxidized fluids are necessary to account for an economic deposit from both genetic models. However, there exists divergence in the sources of these fluids, the paths they follow, the sources of metals, and the possible traps. Barton and Johnson 1996, 2000, noted that heat source and many of the solutes are generally provided by igneous bodies but compositional and petrotectonic controls would be relatively unimportant, whereas larger-scale plumbing systems and paleogeographic controls that favour external brines would be critical. The lack of substantial evaporitic sequences in some IOCG districts has resulted to some school of thought (e.g Pollard, 2000) that strongly question the importance of these sequences as a requirement for the formation of IOCG deposits. They are rather in line with a magmatic source for the ore-forming fluids as well as metals. Mark et al. (2000) proposed a scenario for deposits within the IOCG group ranging from relatively low ƭO2, hotter and deeper deposits (e.g. Ernest Henry) to those forming higher in the crust from more oxidized lower temperature fluids (e.g. Olympic Dam) and that fluid mixing could be the cause of the diversity. Barton & Johnson (2004) came out with a summary of the possible models for IOCG mineralisation based on principal fluid sources (Fig 2).. 9 Mineral texture and composition of magnetite in the Sahavaara IOCG deposit, Pajala area -. A mineral processing approach.
(17) Figure 2: Conceptual illustration of flow paths and hydrothermal features for alternative models for IOCG deposits (Barton, M.D. and Johnson, D.A., 2004).. 10 Mineral texture and composition of magnetite in the Sahavaara IOCG deposit, Pajala area -. A mineral processing approach.
(18) Table2: Synopsis of alternative genetic models for IOCG systems (cf. Figure 1). Fluid Source Fundamental Processes. Magmatic Release of S=-poor metalbearing brine from magma; rise by buoyancy Cooling, wall-rock reaction ± fluid mixing provide trap. Igneous associations. High-K, oxidized suites ranging in composition from diorites to granites Carbonatite and strongly alkaline connections proposed by some. Hydrothermal alteration in feldspathic hosts. Na(Ca) and other types (K, H+) link to magmas Regional Na(Ca) coincident but not directly related to Cu(-Au) Some Fe-oxides with Cu (- Au), may be deeper or higher-T equivalents Barren Fe oxides may form from distinct fluids and commonly in older hydrothermal systems in same area Shallow to mid crustal levels; commonly along regional structures but near causative intrusions Arcs or extensional environments that produce characteristic. Relationship of Fe-oxides to Cu(-Au). Local setting: depth / structure. Global setting. Non-magmatic Basin / surface Metamorphic Thermal convection Metamorphic release of non-magmatic of brine components brines; wall rock by devolatilization or reaction provides reaction with other metals aqueous fluids; rise Cooling, wall-rock by buoyancy reaction or fluid Cooling, wall-rock mixing provide trap; reaction ± fluid second fluid may mixing provide trap provide metals Igneous rocks diverse No necessary (gabbro to granite); connection, though nonmagmatic commonly present examples known Could be heat source Key heat source in in some settings most Can be material Material source, source diversity reflected in geochemistry K (type I), H+ ± Na Primarily K and H+ (Ca) in upwelling alteration associated zones with deposits Na(Ca) ± K (type II) Regional Na(Ca) in recharge zones association reflects sources Mt-rich are deeper, earlier, higher-T parts of ore forming; Mt or Hm also typical with Cu Barren Fe oxides represent lack of S trap for Cu or lack of second Cu-bearing fluid In (mainly) brittle upper crust; plumbing provided by regional or volcanotectonic structures Regions with appropriate brine sources (arid settings or older Cl-rich. Fe-oxides present, but relatively minor (Bi or Chl common); Fe oxides commonly generated by breakdown of mafic minerals rather than Fe introduction. Mid- to shallow crustal levels near or on major structures ; surface fluids require shallow levels Regions with Cl-rich low to intermediategrade source rocks; compressional setting 11. Mineral texture and composition of magnetite in the Sahavaara IOCG deposit, Pajala area -. A mineral processing approach.
(19) magmas (oxidized high-K or alkaline) Key references. Hauck (1989), Pollard (2000), Groves and Vielreicher (2001). materials), plumbing systems, and thermal drives Barton and Johnson (1996, 2000), Haynes et al. (1995), Haynes (2000). (e.g., basin-collapse) or prograde metamorphism Williams (1994), De Jong et al. (1998), Hitzman (2000). 3-d) Exploration Implications Identification of basins, anorogenic magmatic provinces, or subduction-related continental margins containing dominantly oxidised package of rocks including significant source rocks (sub-aerial mafic volcanic rocks and/or continental red beds) and, ideally marine or lacustrine evaporates. The presence of large volumes of magmatic rocks including evidence that magmatism was related to mantle- underplating The presence of magnetite-apatite systems (which are more common than IOCG systems) indicates the correct tectonic setting and is an extremely favourable indicator. Iron oxide-Cu-Au systems will be stratigraphically higher or temporally later, than the magnetite apatite deposits Delineation of high-angle structures related to deep-seated faults. Splays of major faults or regionally sub-parallel fault zones in the hanging walls of major faults appear to be the best target areas Identifications of zones of sodic-calcic, potassic, or hydrolytic alterations which are associated with iron oxide-Cu-Au deposits. In high-level systems, search for evidence of convection systems that involve alkaline, high-salinity lacustrine fluids.. I.4 Area of study and Exploration history 4-a) Location of study area The project area is situated at latitude 67 degrees 22 minutes and longitude 23 degrees 17 minutes east of the eastern flank of Stora Sahavaara hill, about 2 km from the small rural community of Kaunisvaara (Figure 3) some 30 kilometres north of Pajala in the province of Norrbotten-Northern-Sweden, in the region of Norrland, and extends into Swedish Lappland (Fig. 4). The project is located about 5 km south of the Finnish border of the Kolari district. The Payala and the Kolari districts are located on either sides of the international boundary (the Muonionjoki) between the two countries (Sweden and Finland). Several iron deposits considered to belong to the IOCG deposit type are hosted by these districts.. 12 Mineral texture and composition of magnetite in the Sahavaara IOCG deposit, Pajala area -. A mineral processing approach.
(20) Fig 3: Localisation of the Stora- and Södra Sahavaara magnetite deposit in the Kaunisvaara iron field.. 13 Mineral texture and composition of magnetite in the Sahavaara IOCG deposit, Pajala area -. A mineral processing approach.
(21) Fig4: location of project area.. 4-b) Exploration history and Review of previous work in the area Exploration history Since the start of exploration in the Payala and Kolari districts covering a total surface area of 1,600 square kilometres, 30 magnetite bodies have been identified of which 20 lies within the Kolari District of Finland and ten within the Pajala district of Sweden. Stora Sahavaara is the largest of the known magnetite deposits within the Pajala district, and extensive work is 14 Mineral texture and composition of magnetite in the Sahavaara IOCG deposit, Pajala area -. A mineral processing approach.
(22) currently being performed on this deposit. The ore bodies of the once referred Payala ironstone belt are today collectively known as the Kaunisvaara Field. One of the earliest studies on the Kaunisvaara field was by V. Tanner. In 1918, applying a dip needle prospecting technique he discovered five ore bodies; Palotieva, Norra Tapuli, Stora Tapuli, Stora Sahavaara, and Södra Sahavaara (Lundberg, 1967). The Swedish Geological survey (SGU) and other companies then realised the important riches of the Kaunisvaara field and this foster exploration. The next success came in 1949 when Boliden AB discovered the Ruutijärvi ore body which was closely followed by the SGU credited discovery of a faulted extension of this deposit called `Blind Kuutijärvi`. It didn`t ended here as successes led to the hunt for more discoveries. SGU worked closely with the Johnson and Co in 1958 and the fruits of this partnership were the Karhujärvi and Suksivuoma magnetite lenses further south of the Kaunisvaara Iron Ore Field. In 2000 Anglo acquired the Payala property and a GeoTem survey was flown over parts of the current license area. Northland Resources Inc in 2004 entered into a letter of agreement with a wholly owned subsidiary of Anglo American Exploration plc (Anglo) to acquire a 100 percent interest in its ―Swedish Pajala Properties‖ which includes the Stora Sahavaara magnetite deposit. Initial resource delineation drilling commenced at Stora Sahavaara in 1960 and continued for five years during which 46 drill holes were completed for 11,798 meters. Drilling by LKAB then increase the number of drill holes to 50 in 1980s for a total of 12,018 meters. Northland carried out further drilling and increases the drill holes to 60 totalling 14,222 meters, which they used to estimation the iron-copper resource. Presently the Sahavaara deposit is at an advance stage pending production.. Review of previous work The earliest piece of work in the Kaunisvaara iron field was in 1918 by V. Tanner during which he discovered five ore bodies. Since then the Kaunisvaara Ore Field has been described in numerous geological reports by the SGU (Eriksson 1955, Fredrikson 1986, Frietsch 1957, 1962a, 1962b). Lundberg (1963) carried out detailed geological mapping of the area. These geological maps were updated by Padget (1977) and Witschard (1984, 1986). Heavy mineral stream sediment-sampling programme during 1973 and 1974 was conducted by Bergström on a regional scale (Bergström 1975). Magnusson (1984) created a statistical evaluation of the data from stream sediment sampling and peat sampling. Ronkko (1985) then further compiled all of the previous peat and stream sampling at a scale of 1:50,000 displaying Cu-Mo, Pb-Zn, Co-Ni and Fe-Mn concentrations. Hanson (1984) and Nilsson (1991) carried out extensive boulder and base of till sampling on a regional-scale. Danielson (1982) tested samples from Stora Sahavaara and Tapuli for cobalt but found no anomalous results. Carlson and his colleagues tested seven iron occurrences for gold and found that samples from Tapuli produced anomalous results (Carlson et al 1982). Extensive deep till and bog has made geophysical survey in this region very important and it is use as the principal exploration method especially in early reconnaissance survey. Airborne magnetometer survey in the late 1940’s and 1958 by Boliden AB and Rederi AB Nordstjernan detected mineralisation at Ruutijärvi and Suksivuoma respectively (Frietsch, 1997). Ground base magnetic data existed at Stora Sahavaara since 1960 when a torsion magnetometer was used as an exploration tool with 100-meter line spacing and 20-40 meters between stations covering 1.4 square kilometers. At this time, gravimetric measurements were also conducted 15 Mineral texture and composition of magnetite in the Sahavaara IOCG deposit, Pajala area -. A mineral processing approach.
(23) along several cross lines to determine the strike of the magnetite body (Lundberg 1965). Sixty five (65) new target-areas were identified by Lindberg (1990) by use of digital imaging to analyze airborne magnetic, slingram and gravity data for the Pajala district. A lot of work is still needed for the effective classification of the iron ores of the Kaunisvaara Field, most especially as recent research is turning the table around from an earlier considered skarn type deposits to a more recent IOCG style of mineralisation.. I.5 Regional Geology In northern Sweden there were several periods of mineralization associated with the Svecofennian (also called Svecokarelian) orogeny that took place about 1900 to 1750 million years ago. The mineralization is associated with pulses of metamorphism and magmatism after plate amalgamation. The results are large deposits of copper, iron, and gold. Identification of the tectonic and metamorphic environment during the 150 million-years-long Svecofennian orogenic event is critical information for targeting mineral exploration. Published and unpublished data on northern Fennoscandia indicate multiphase Palaeoproterozoic hydrothermal mineralization during a period of 160 m.y. (Mänttäri, 1995; Billström and Martinsson, 2000; Niiranen et al., submitted; Niiranen et al., in prep.; Wanhainen et al., in prep.). This further implies that mineralization is linked to different tectonic settings and with different magmatic suites. Unlike most other shield areas, the Fennoscandian shield is more mineralized in the Palaeoproterozoic compared to the Archaean. At about 2.5-2.0 Ga (early Palaeoproterozoic), extensional events resulted to the deposition of clastic sediments, intercalated with volcanic rocks (greenstones) on the deformed and metamorphosed Archaean basement. These Svecofennian supercrustal sequences were put in place during the svecokarelian orogeny in the palaeoproterozoic (Kathol and Weihed, 2005, Bark and Weihed, 2007). Svecofennian subduction generated calc-alkaline volcanic rocks and related volcanoclastic sedimentary units were deposited around 1.9 Ga in the northern Fennoscandia in a subaerial to shallow water environment. The Svecofennian volcanic rocks form hosts to apatite-iron ores and various styles of epigenetic Cu-Au occurrences. The Palaeoproterozoic volcanic and sedimentary rocks were deformed and metamorphosed during the Svecokarelian Orogeny (1.95–1.77 Ga) contemporaneous with the emplacement of several generations of mafic to felsic intrusions. Large-scale migration of saline fluids during the many stages of igneous activity, metamorphism and deformation is expressed by regional scapolitization, albitization and albite-carbonate alteration in the region. Scapolitization has been suggested to be related to felsic intrusions (Ödman, 1957), or to be an expression of mobilized evaporites from the base of the greenstone successions during metamorphism (Tuisku, 1985; Frietsch et al., 1997). The geology of northern Sweden can be divided into four principal tectonic units: (1) Archaean units, (2) Paleoproterozoic units with Archaean basement (Karelian Craton), (3) Juvenile Paleoproterozoic units without any known Archean basement, and (4) Caledonian rocks (Weihed, 2004). Achaean units (composing mainly of metagranitoids which has 16 Mineral texture and composition of magnetite in the Sahavaara IOCG deposit, Pajala area -. A mineral processing approach.
(24) intruded older supracrustal rocks) lack any significant mineralization and are only locally exposed. The unit of interest is the Paleoproterozoic rocks with a valued metallogenic importance, and known not only to constitute a characteristic unit for the IOCG deposit type but also acting as the principal host to several deposit types consider to be end members of the IOCG deposit family. Particular formations of interest are: the metavolcanic rocks (1.91-1.88 Ga. Porphyry group), the metasedimentary and metabasalt (1.96-1.85 Ga. Svecofennian), and the metasupercrustal rocks, metagabbro, metadiabase (2.4-1.196 Ga. Karelian). Before looking into the detail stratigraphy of the different rock suites, first let us consider some of the reasons why this area is a suitable exploration target for the IOCG deposit type. The Archaean craton is known to have undergone some rifting events during the late Achaean or earliest Proterozoic (at about a 2.44 Ga.). Remnants of these rifting events are marked by the mafic to ultramafic intrusions and dike swarms (Martinsson, 1997). At about 2.4–1.96 Ga. (Karelian) subsequent sedimentation, volcanism and magmatism took place in the rift basins and the development of classical greenstone belts. The Svecofennian (1.96 to 1.85 Ga) supracrustal rocks have overlain these units. The Svecofennian rocks are generally considered to be related to convergent tectonics and accretionary processes along the old continental margin (Weihed, 2004). Bergman et al., 2001 grouped the oldest Karelian rocks to the Kovo Group and comparable units in northernmost Sweden. The petrology of these groups of rocks includes the 2.39 to 2.33 Ga. metasedimentary and mafic to intermediate metavolcanic rocks interpreted by Kumpulainen (2000) as deposited at the margin of an early Proterozoic marine rift basin. Further works by Martinsson, 1997, in the Kiruna area shows that the basaltic rocks are tholeiitic and the volcaniclastic calc-alkaline. The Greenstone group of rocks with main rock types according to Bergman et al. (2001) being metabasalts, graphite-bearing metaargillite, carbonate rocks, and ultramafic rocks are found in several areas in northernmost Sweden (Fig 5). Martinsson, 1997 suggested a MORBor LKT-like character for these rocks. He equally noted the low stratigraphic position of komatiites in the Kiruna area (Martinsson, 1997). Other rocks occurring in this area are volcaniclastic units, commonly intercalated with carbonate rocks, black schist, iron formations, and chert. There is no regional unconformity defining the arbitrary transition considered to mark the changes from divergent to convergent plate movements from Karelian to Svecofennian rocks within the area underlain by an Archaean basement (Weihed, 2004). This further complicates the tectonic variation. Nonetheless, the convergent plate movement is related to the Svecokarelian orogeny and accretionary processes. In parts of the Kiruna area the transition is marked by local unconformities or disconformities. Offerberg, (1967) and Martinsson and Perdahl, 1993, 1995 classified the metavolcanic rocks in the Kiruna area into the lower Porphyrite group consisting of low Ti andesites, basalts and minor felsic volcanic rocks (Bergman et al., 2001) characteristically with large plagioclase phenocrysts, and the upper Porphyry group, varying from basalt to rhyolite in composition, with some few volcaniclastic rocks. Martinsson and Perdahl (1995) concluded that the former was formed as calc-alkaline volcanic rocks in a compressional environment while the later was formed in an extensional environment.. 17 Mineral texture and composition of magnetite in the Sahavaara IOCG deposit, Pajala area -. A mineral processing approach.
(25) Fig 5: Distribution of major rock units in Northern Sweden (Wanhainen et al., 2006).. I.6 Deposit (district) Geology The Pajala ore district is hosting about ten (10) magnetite deposits specifically in the Kaunisvaara field. Deposits of similar style occur and have been well studied on the Finnish side of the Lappland. In the Pajala district these deposits are located within the Pajala Shear Zone (PSZ), a regional structure locally concordant with bedding and forms part of the BalticBothnian Mega-shear, a 50 to 100 kilometre wide system of thrust and reverse faults, which are considered to have been active during the Svecofennian Orogeny at about 1.85 Ga. (fig 6) (Weihed, 2004). They are hosted by metasedimentary rocks; quartzites, schists, phyllites and dolomites overlying the Greenstone group which is the oldest Paleoproterozoic (1.9 Ga) unit of the region. The greenstone unit also consist of volcanic (pyroclastic) rocks such as tuff, agglomerate, and amphibolites. Lithologies strike N25°E and dip 60°W and the stratigraphic sequence ages downward respecting the law of superposition in undisturbed sequences. The geology of the Stora Sahavaara deposit can be easily studied by dividing the rocks into subassemblages such as the hangingwall assemblage, the ore zone, and the footwall assemblage, since the different rock units lies almost entirely concordant to each other and with respect to the ore body, with little and/or no noticeable displacement.. 18 Mineral texture and composition of magnetite in the Sahavaara IOCG deposit, Pajala area -. A mineral processing approach.
(26) The hanging-wall assemblage is to the west of the ore zone and it consists of very thick and continuous (regular) rhythmic sequences of light-grey moderately biotite-bearing quartzite and a dark-grey biotite-rich quartzitic phyllite (fig 7). Other accessory minerals of this unit include feldspars, muscovite, magnetite and the low temperature and pressure metamorphic mineral zoisite. Microscopy of thin sections reveals a heterogranoblastic texture. Isoclinal folds of centimetre-scale are typical in the phyllite. The quartz-phyllitic layers in particular are frequently micro-folded, flow-folded or entirely transformed to granitic gneiss by granitisation (Lundberg, 1967). Close to the ore zone the quartz, feldspar and biotite relationship becomes inversely proportional. The quartz content increases as feldspar and biotite decrease in abundance. The immediate wall rock to the skarn-zone is typically a finegrained quartzite. The beds of this hanging-wall unit have an average thickness of 65 m. The western limit of this unit is not yet accurately known.. Fig 6: Regional Geology and location of Magnetite Deposits in the Pajala District. Note the link of the deposits to major structural systems. (Northland`s Technical report, 2006). The Sahavaara ore consist principally of magnetite and some skarn, though hematite has been reported in other deposits as a result of hydrolysis (weathering processes). Copper concentrations are also more or less elevated. The most prominent sulphide minerals are pyrrhotite and pyrite. Mg-rich skarn with serpentine as the dominant mineral and likewise diopside, tremolite and phlogopite constitute the gangue. The tabular, slightly folded and largely regular ore body is concordant to the rocks of both the hanging and the foot walls assemblages (Fig 7). Adjacent wall rocks are highly faulted and vary in width from one centimetre to twenty meters. Two distinct fault orientations are identified; a NNE-SSW direction in accordance with the strike of metamorphic foliation and a second which strikes 19 Mineral texture and composition of magnetite in the Sahavaara IOCG deposit, Pajala area -. A mineral processing approach.
(27) NW-SE with steep to moderate dips (Lindroos 1974). Contacts between the host rocks, skarn and mineralization are mainly tectonic. Calcite veinlets are common throughout the deposit. The Sahavaara magnetite body sandwiches the hanging-wall quartzite and footwall graphitic schist and skarn-scapolitefels. The original protolith is depicted by minor remnants of skarnaltered dolomite occurring within the deposit. The Sahavaara main body extends continuously over a strike length of 1,400 meters with an average true width of 52 meters. Three principal skarn types; clinopyroxene-amphibole skarn, consisting of equal proportions of tremolite and diopside with very limited amounts of scapolite, magnetite and garnet, and serpentine skarns; consisting dominantly of very fine-grained serpentine with varying amounts of magnetite, chlorite, talc and calcite are noticed to have enclosed the ore and at some depths occur within the ore. Minor amounts of scapolite skarn consisting dominantly of fine to medium-grained scapolite with varying amounts of diopside and tremolite is however noted. The clinopyroxene-amphibole skarn is defined by a zone between the hanging wall assemblage (quartzite) and the ore body and where it occur within the ore, it often occurs as large magnetite-free aggregates. However, these skarn types scarcely occur within the magnetite body. On the other hand, the serpentine skarn is common in both the hanging wall and footwall assemblages and constitute the gangue of the ore. The scapolite skarn is seldom observed in the hanging wall unit but do largely occur in the footwall unit. Graphitic schist with isoclinal folds of centimetre-scale and consisting of fine-grained quartz, scapolite, graphite, biotite, and tremolite, and accessory sphene and apatite is the footwall rock. This unit is rich in pyrrhotite and lesser amounts of pyrite with some local chalcopyrite mineralization. East of the graphitic schist is a 150-200 meter thick unit of phyllite that contains a 30-40 meter wide intercalation of dolomitic marble. The lowest unit in the stratigraphic sequence further east is a mafic metavolcanic rock (Fig 7). It is known as the volcanic greenstone group and consists of rock types such as tuffs, agglomerate, and amphibolites. Meta-diabase dykes are abundant throughout the mineralized sequence. These dykes occur parallel to the NW-SE oriented faults and shears and are younger than both the skarn and the magnetite mineralization as well as the Lina Suite granites (1.82-1.79 Ga) peripheral to the deposit (Lindroos, 1974). The dykes are consistently altered forming scapolite- or K-feldspar (Lundberg, 1967) Previous views had considered the Stora Sahavaara iron occurrence as a broad group of magnetite-dominated, Ca-Mg- and Mg-silicate skarn-hosted deposits which occur throughout northern Sweden within a Karelian volcano-sedimentary domain. Several deposits considered to be of same style also occur and have been well studied at the Finnish part of the Lapland, in the Kolari district. These deposits identified to be epigenetic Fe-Cu +Au deposits occur within the same structures as the Stora Sahavaara deposit. Niiranen, 2005; Niiranen et al., 2006, recently suggested these deposits to belong to the increasingly important group of iron-oxidecopper-gold (IOCG) deposits.. 20 Mineral texture and composition of magnetite in the Sahavaara IOCG deposit, Pajala area -. A mineral processing approach.
(28) Fig 7: Deposit geology and drillhole locations. (Northland`s Technical report, 2006) 21 Mineral texture and composition of magnetite in the Sahavaara IOCG deposit, Pajala area -. A mineral processing approach.
(29) CHAPTER II: PETROGRAPHIC AND MINERALOGICAL DESCRIPTIONS This chapter gives a detailed summary of the macroscopic and microscopic descriptions of the rocks of both the hanging- and footwall and the ore unit based on the drillcores considered in this work. For a broader description, the reader is referred to appendix D. After the drill cores were logged some sections were further studied in hand specimens and thin sections were made from samples considered relevant to better describe the compositional and textural variations in time and space. The polished thin sections were examined using a polarising microscope.. II.1 Macroscopic observations The hangingwall rocks of the northern terminal are generally leucocratic and fine-grained in texture. However, they vary in composition and character (SAH63008, SAH65005, SAH05004, and SAH05011, Appendix D-1). The rocks have suffered from variable degree of metamorphism and granitisation, and are often characterised by quartzofeldspathic bands and/or ptygmatic structures. They are quartz, pyroxene, amphibole, arfvedsonite, and serpentine bearing. The later is mainly common at the border of the ore zone. Generally pyroxene and amphibole skarns dominate at the hangingwall. Tremolite and serpentine contents increase progressively towards the ore zone. Carbonate units are also noted. The rocks of the hangingwall are dominantly quartzite (SAH65005, SAH05011) and siltstone (SAH63008) (Appendix D-1). The footwall rocks are rich in sulphides and the dominant rock types are graphitic schist and phyllite. The ore of the northern terminal is dominantly fine- to coarse-grained. Only minor intercalations of magnetite poor skarn occur within the ore and this is dominantly the serpentine skarn (Fig 8). Details on how the ore zone varies in composition, texture, and character are given in appendix D-1. a ). b ). 22 Mineral texture and composition of magnetite in the Sahavaara IOCG deposit, Pajala area -. A mineral processing approach.
(30) c ). d ). Fig. 8: a) alteration and weathering of hangingwall rock, b) rich ore, massive and strongly magnetic with limited coarse-grained amphiboles and sulphides at 55.25 m to 61.60 m ( SAH05004), c) distinct limit between ore and skarn, d) alternation of pyroxene and serpentine skarns. Similar rocks occur at the hanging- and footwall units of the central zone as those of the northern terminal, however, they vary in character and are weakly granitised (Fig 9). These rocks include quartzite and/or quartzitic phyllite at the hanging wall, and graphitic schist and marble (carbonates) at the footwall. One important observation is the general increase in carbonates most especially around the ore zone, and an increase in chalcopyrite and valleriite contents as well in the ore zone. Serpentine skarns dominates over the pyroxene and amphibole skarn types. Small amounts of biotite skarn are also present. Another aspect of the central zone is the regular occurrence of mafic intrusions. Note the increase in Ccp content from drillcore SAH05014 at the south of the central zone to drillcore SAH05012 towards the north (appendix D-1). The ore character and composition vary considerably from a coarsegrained with disseminated sulphide via a massive ore to a more banded stye (appendixD-1). a ). b ). c ). d ). 23 Mineral texture and composition of magnetite in the Sahavaara IOCG deposit, Pajala area -. A mineral processing approach.
(31) Fig. 9: a) weakly granitised quarzitic phyllite, b) ore from SAH05012, c) ore from SAH05014, d) serpentine-amphibole-pyroxene skarn. At the southern terminal (SAH63021), the stratigraphy becomes more rhythmic and the ore zone is narrow. The hangingwall rocks to the Södra ore lens are albitic-tuffite and are whitish red in colour, altered, and more or less porphyroblastic in texture. The predominant minerals are quartz, carbonates and graphite. At the hanging wall of the minor ore lens the lithology changes upwards from serpentine skarn intercalated in some places with thin carbonate units, to tuffite. The graphite-schist and tuffite are in a rhythmic sequence at the footwall and part of the hangingwall. At the foot wall zone the mineral bands of the tuffite appears mixed, though some small striations of the greenish band and carbonates are observed (appendix D-1). There are differences in composition of the tuffites occurring at the hangingwall unit compared to those at the footwall. Their composition also varies along the ore lens. The tuffites occurring at the footwall have a higher and fairly constant Fe2O3 concentration as compared to those at the hangingwall. The hanging wall of the Södra Sahavaara deposit is constituted of scapolite bearing and layered phyllite. Carbonates and weakly granitise metadiabase are also present (Fig 10). The skarn is dominated by amphibole and pyroxene. Serpentine skarn units are subordinant (SAH07019, appendix D-1).. Fig. 10: Magnetite ore from the SAH07019 drillcore of the Södra Sahavaara deposit. Note the distinct limit with carbonate rocks.. II.2 Microscopic observations 2-a) Foot and Hanging wall Rocks:. SAH63008 Microscopic observation of thin section from SAH63008 at 150m depth (hangingwall) shows a grano-lepido-porphyroblastic texture (Fig 11a). The main minerals present are quartz, feldspars (both plagioclase and potassic) and pyroxene. Serpentine occur in minor amount in 24 Mineral texture and composition of magnetite in the Sahavaara IOCG deposit, Pajala area -. A mineral processing approach.
(32) regions with large pyroxene concentrations. The rock is generally altered. The pyroxenes can be seen to have occurred in two generations: the first generation of crystals are porphyroblastic. These porphyroblast are highly altered and in most places just relics are left. The alteration product here is serpentine. The needle-like (acicular) crystals considered to be of a second generation are less altered. However, some are mark by alteration rims (appendix D-2). At 51m depth, the thin section shows a granolepidoblastic texture. Pyroxenes, quartz, plagioclase, and microcline are the main minerals present (appendix D-2). Opaque minerals are also noted. Crystals of both the pyroxenes and plagioclase exhibit zoning. These two minerals appear separated in some parts and more or less mixed in others with the pyroxenes crystals elongated in a particular plane (fig 11b). a ). b ). Fig 11: a) photomicrograph showing the general presentation of the rock at 150m depth of SAH63008 drillhole, Sahavaara deposit. Notice the first generation pyroxenes relicts at the top right corner, b) Photomicrographs showing deform nature of the minerals characterising the melanosome layers of the rocks at 51m depth of the SAH63008 section of Sahavaara deposit. SAH65005 Thin section from the footwall rocks at 123.3 m depth presents a porphyroblastic texture. The porphyroblast consists of plagioclase, pyroxenes, and amphiboles, and are distributed around or with graphite. The second phase, the groundmass, is dominated by plagioclase feldspar. Graphitie dissemination of a possibly younger generation can be seen to traverse the rock in two directions (fig 12). At 52.5m, the thin section reveals a characteristic mesh texture which is a common feature of serpentine (fig 12b). The magnetite seems to have been formed in two stages; the first stage during the complete alteration of tremolite (-actinolite) to serpentine and then the later stage from the partial alteration of serpentine. Serpentine makes up more than 50 percent of the mineral composition. Under Plane polarise light (PPL), large incorporation of tremolite, calcite, and chlorite into the oxide phase is observed in veins. The light-coloured tremolite reveals that it is enriched in Mg and deficient in Fe.. 25 Mineral texture and composition of magnetite in the Sahavaara IOCG deposit, Pajala area -. A mineral processing approach.
(33) b ). a ). Fig 12: Concentration of porphyroblast along and within graphite dissemination in two characteristic direction, b) Photomicrographs of serpentine with characteristic mesh texture. Relicts of primary minerals can be noted. This thin section from the hangingwall skarn at 21 m depth shows a porphyroblastic texture (fig 13a). All minerals occur both as porphyroblasts and in the groundmass. The main minerals present include; pyroxene (diopside +/- enstatite), tremolite (amphibole) and arfvedsonite. These minerals are more or less unaltered. Pyroxenes constitute more than 50% of the mineral composition. The rock at 15 m depth is heterogranoblastic in texture (fig 13b). Quartz makes up more than 50 percent of the mineral constituent and exhibit undulose extinction. It occurs as irregular grains and appears unaltered. The quartz occurs in some areas mostly as coarse-grained of up to 1 mm in diameter. Hornblende and arfvedsonite occurs as highly altered porphyroblasts, and in most cases just relics are left. b ). a ). Fig13: Photomicrographes a) showing porphyritic texture of pyroxene skarn, b) Heterogranoblastic texture of quartz.. 26 Mineral texture and composition of magnetite in the Sahavaara IOCG deposit, Pajala area -. A mineral processing approach.
(34) SAH63021 Thin section from the footwall rocks at 60 m shows a grano-lepido-porphyroblatic texture. The minerals present are quartz, carbonates, amphiboles (hornblende), graphite, cordierite, and anthophyllite (fig 14a). Carbonates, amphiboles, and graphite are the principal porphyroblasts and commonly intergrowth together. Quartz is more concentrated in the groundmass. The minerals have undergone some degree of alteration. The quartz grains are more or less deformed (fig 14a). At 79.5 m, the rocks are intensely altered. The main minerals identified are arfvedsonite (pyroxenes and amphiboles), graphite, calcite, and scapolite. Relics of altered porphyroblasts are common along graphite (fig 14b) and intergrowth with the graphite. Graphite is equally very disseminated in the rock, and constitute about 40 percent or so of the rock`s composition.. a ). b ). Fig14: a) carbonate veins and deformed nature of rock, b) relics of altered porphyroblasts in graphite. At 140 m depth, at the hangingwall of the Södra oelens, the rock is banded and main minerals are biotite, clinopyroxene, tremolite, and some quartz. Biotite occurs as tabular crystals exclusively in the darker band. The tabular biotite crystals are aligned towards a particular direction (deformation) (fig 15a). Pyroxenes and tremolite makes up more than 50 percent of the mineral content and are intensely altered. They are mainly granoblastic. The biotite is altered as well and characterised by alteration rims. The rocks at 241.5 m depth are porphyroblastic in texture. The major minerals are biotite, tremolite, pyroxenes, chlorite, and minor amounts of quartz and apatite. Most of the tremolite and pyroxenes occurs as acicular crystals. The porphyroblasts of these minerals are corroded, altered and zone, and some show visible cleavages. These two minerals constitute the majority of the rock composition. Chlorite is limited to areas of intense alteration and where serpentine is being form (fig 15b). Graphite occurs disseminated.. 27 Mineral texture and composition of magnetite in the Sahavaara IOCG deposit, Pajala area -. A mineral processing approach.
(35) a ). b ). Fig15: Photomicrographes showing; (a) Preferential alignment of acicular crystals, b) formation of serpentine from pre-existing silicates alteration.. II.3 Microscopy of Ding Davies Tube (DDT) Test Buttons The aim of the DDT microscopy is to correlate the DDT test result of both the concentrate and the tailings with the microprobe assays as well as with basic optical mineralogy of the ore and associated lithologies. There are few cases where the concentrate is poor. Thirty nine (39) DDT test buttons from drill sections have been studied by light microscopy and the findings are as follows: (EM stands for the non-magnetic phase (Tailings) and M for the magnetic phase (concentrate)). Note that only a summary of the DDT test results is given here. A more detail case specific study is included in appendix D-3. DDT test buttons from drillcores SAH05011 and SAH05004 were use in the study of the northern terminal. The microscopy of these buttons shows variation in the test concentrate both vertivally and horizontally. Concentrate from the upper levels are high in MgO and with restricted sulphide content (little and/or no pyrrhotite). Some of the magnetite grains are corroded, altered, zoned and locked within silicates, and in most cases the silicates characterise the main frame work. Intergrowth and incomplete segregation of magnetite from silicates are also common. Critical examination of some of the magnetite-like grains in the nonmagnetic fraction reveals that they are magnetite pseudomorphs after silicates. At mid levels pyrrhotite and silicate contents increases. Pyrrhotite grains in the nonmagnetic fraction are finer. There is little and/or no pure magnetite grains in the tailings. However, there exist magnetite pseudomorphs after silicates. In the magnetic concentrate silicate (serpentine) rim grains, and also occur as infill and/or inclusions in corroded magnetite grains. No magnetite pseudomorphs after silicates were identified. The principal minerals in the concentrate are magnetite and pyrrhotite. There is horizontal variation in the concentrate mineralogy at deeper levels. At SAH05011, sulphides are very limited and if present it is mainly pyrite, while at SAH05004 sulphide are common both in the concentrate and tailings, and are mainly pyrrhotite and chalcopyrite. Magnetite pseudomorphs after silicates are also present but restricted to the nonmagnetic fractions. 28 Mineral texture and composition of magnetite in the Sahavaara IOCG deposit, Pajala area -. A mineral processing approach.
Figure
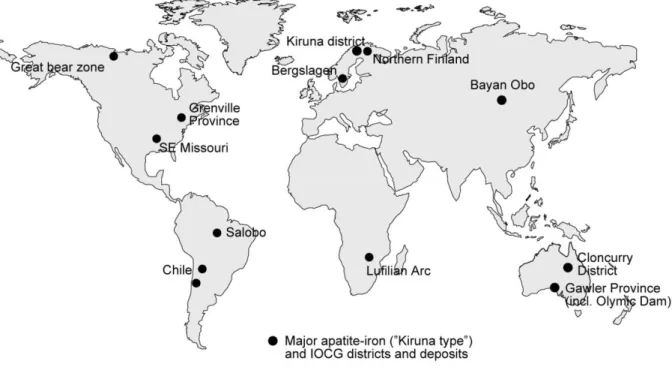
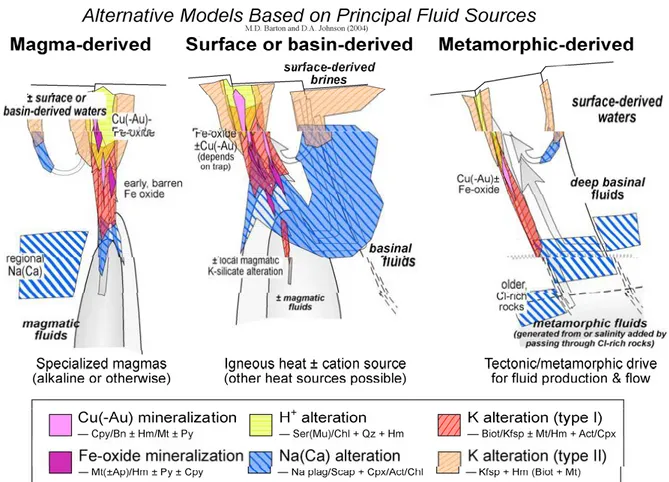
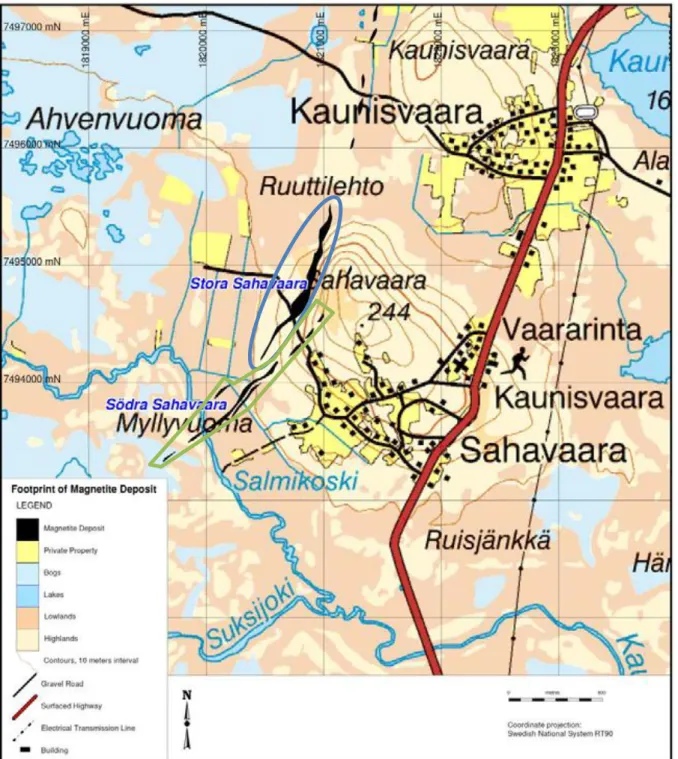
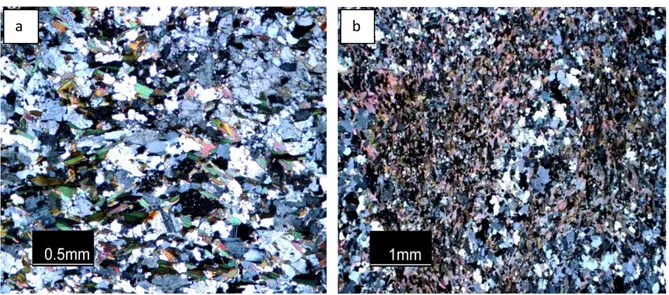
Related documents
Different mineral processing plants were analysed in detail regarding the energy consumption.. For a plant like Aitik with low grade copper ore the comminution, especially
46 Konkreta exempel skulle kunna vara främjandeinsatser för affärsänglar/affärsängelnätverk, skapa arenor där aktörer från utbuds- och efterfrågesidan kan mötas eller
This project focuses on the possible impact of (collaborative and non-collaborative) R&D grants on technological and industrial diversification in regions, while controlling
Analysen visar också att FoU-bidrag med krav på samverkan i högre grad än när det inte är ett krav, ökar regioners benägenhet att diversifiera till nya branscher och
För att uppskatta den totala effekten av reformerna måste dock hänsyn tas till såväl samt- liga priseffekter som sammansättningseffekter, till följd av ökad försäljningsandel
a) Inom den regionala utvecklingen betonas allt oftare betydelsen av de kvalitativa faktorerna och kunnandet. En kvalitativ faktor är samarbetet mellan de olika
Parallellmarknader innebär dock inte en drivkraft för en grön omställning Ökad andel direktförsäljning räddar många lokala producenter och kan tyckas utgöra en drivkraft
Industrial Emissions Directive, supplemented by horizontal legislation (e.g., Framework Directives on Waste and Water, Emissions Trading System, etc) and guidance on operating